Peroxiredoxin hyperoxidation increases in the presence of bicarbonate/carbon dioxide
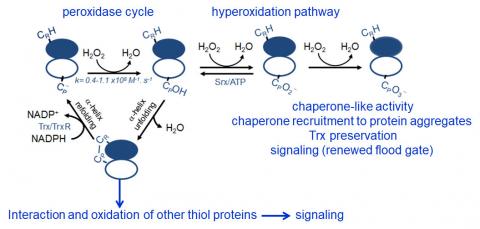
Highlights by Daniela R. Truzzi and Ohara Augusto, from Instituto de Química da USP
Corresponding author e-mail: dtruz zi @iq.usp.br
Peroxiredoxins (Prx) are abundant thiol peroxidases that react rapidly with H2O2, constituting an important antioxidant defense and acting as sensors and transmitters of H2O2 signals in cells. Eukariotic 2-Cys Prxs lose their peroxidase activity at high hydroperoxide levels in a process called hyperoxidation, which constitutes a pathway to Prx functions beyond the antioxidant activity (Fig. 1) [1]. Since the biologically ubiquitous HCO3–/CO2 pair accelerates the reaction of H2O2 with several biothiols [2, 3], we examined whether physiological concentrations of the HCO3–/CO2 pair (25 mM) could increase recombinant human peroxiredoxin 1 (Prx1) hyperoxidation by H2O2 [4]. Immunoblotting, kinetic and MS/MS experiments revealed that HCO3–/CO2 increases Prx1 hyperoxidation and inactivation both in the presence of excess H2O2 and during enzymatic (NADPH/thioredoxin reductase/thioredoxin) and chemical (dithiothreitol) turnover. Based on previous studies, we hypothesized that the stimulating effect of HCO3–/CO2 was due to HCO4– (peroxymonocarbonate), a peroxide present in equilibrated solutions of H2O2 and HCO3–/CO2 [2, 3]. Indeed, additional experiments and calculations uncovered that HCO4– oxidizes CPSOH to CPSO2– with a second-order rate constant two orders of magnitude higher than H2O2 ((1.5 ± 0.1) × 10⁵ and (2.9 ± 0.2) × 10³ M⁻¹.s⁻¹, respectively) and that HCO4– is 250 times more efficient than H2O2 at inactivating 1% Prx1 per turnover [4]. The fact that the biologically ubiquitous HCO3–/CO2 pair stimulates Prx1 hyperoxidation may be quite relevant to cell homeostasis because the antioxidant and redox relay functions of the enzyme decline but other actions may rise, such as the chaperone-like activity, the redox signaling pathways mediated by Cys-based proteins that are poorly reactive towards H2O2 and the maintenance of Trx-dependent activities (Fig. 1). Relevantly, parallel studies led by Christine Winterbourn and co-workers reported that the HCO3–/CO2 pair also stimulates Prx2 and Prx3 hyperoxidation [5] and protein tyrosine phosphatase 1B inactivation involved in epidermal growth factormediated signaling [6]. Taking together, these recent studies confirm that HCO4– deserves further investigation as a biological oxidant [3] and point to a possible role of HCO3–/CO2 levels in H2O2mediated signaling.
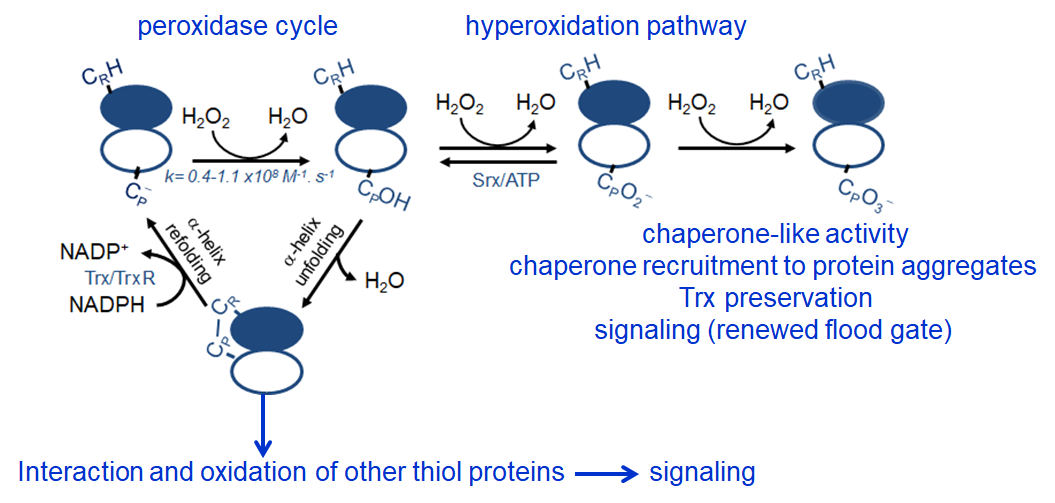
Figure 1. Simplified scheme of the catalytic cycle and the hyperoxidation pathway of 2-Cys Prxs and the activities related to them.
References
- E. A. Veal, Z. E. Underwood, L. E. Tomalin, B. A. Morgan, C. S. Pillay. Hyperoxidation of Peroxiredoxins: Gain or Loss of Function? Antioxidants & Redox Signaling, 28(7): 574–90, 2018. | doi: 10.1089/ars.2017.7214
- D. F. Trindade, G. Cerchiaro, O. Augusto. A Role for Peroxymonocarbonate in the Stimulation of Biothiol Peroxidation by the Bicarbonate/Carbon Dioxide Pair Chemical Research in Toxicology, 19(11): 1475–82, 2006. | doi: 10.1021/tx060146x
- D. R. Truzzi, O. Augusto. Influence of CO2 on Hydroperoxide Metabolism Hydrogen Peroxide Metabolism in Health and Disease, (Vissers, M.C.M., Hampton, M., Kettle, A.J. eds) 81–99, Oxidative stress and disease, Taylor & Francis/CRC Press, Boca Raton, 2017. | doi: 10.1201/9781315154831-4
- D. R. Truzzi, F. R. Coelho, V. Paviani, S. V. Alves, L. E. S. Netto, O. Augusto. The bicarbonate/carbon dioxide pair increases hydrogen peroxide-mediated hyperoxidation of human peroxiredoxin 1 Journal of Biological Chemistry, 294(38): 14055–67, 2019. | doi: 10.1074/jbc.ra119.008825
- A. V. Peskin, P. E. Pace, C. C. Winterbourn. Enhanced hyperoxidation of peroxiredoxin 2 and peroxiredoxin 3 in the presence of bicarbonate/CO2 Free Radical Biology and Medicine, 145: 1–7, 2019. | doi: 10.1016/j.freeradbiomed.2019.09.010
- M. Dagnell, Q. Cheng, S. H. M. Rizvi, P. E. Pace, B. Boivin, C. C. Winterbourn, E. S. J. Arnér. Bicarbonate is essential for protein-tyrosine phosphatase 1B (PTP1B) oxidation and cellular signaling through EGF-triggered phosphorylation cascades Journal of Biological Chemistry, 294(33): 12330–8, 2019. | doi: 10.1074/jbc.ra119.009001
Add new comment